ALPS II
Any Light Particle Search
ALPS II
What is the ALPS II project?
ALPS looks for ALPs
The most famous WISP candidate is the axion, which first was introduced to explain the non-observation of the violation of time reversal invariance in strong interactions and which later turned out to also be a prime candidate for a constituent of the dark matter in the universe. Besides the axion, there are numerous other WISP candidates: so-called axion-like particles (ALPs), "hidden sector photons" or light minicharged particles.
It is therefore an important and fundamental question whether any of these light particles exists. WISP scenarios gain support by recent astrophysical studies like the TeV-photon emission of active galactic nuclei or the properties of white dwarf stars, which hint at the existence of ALPs.
Light shining through a wall
ALPS II is a light-shining through a wall (LSW) experiment to search for WISPs. Potential WISP candidates are axion-like particles or hidden sector photons. Axion-like particles may convert to light (and vice versa) in presence of a magnetic field. Similarly, hidden sector photons "mix" with light independent of any magnetic fields. This is exploited by ALPS II- Light from strong laser is shone into a magnetic field. Laser photons can be converted into a WISPs in front of a light-blocking barrier (production region) and reconverted into photons behind that barrier (regeneration region). The experiment exploits optical resonators for laser power build-up in a large-scale optical cavity to boost the available power for the WISP production as well as their reconversion probability to light.
Quest for a sensible sensitivity
The ALPS I experiment at DESY set the world-wide best laboratory limits for WISPs in 2010, improving previous results by a factor of 10.
After its completion the ALPS collaboration decided to continue looking for WISPs by designing the ALPS II experiment for probing further into regions where there are strong astrophysical hints for their existence.
With the ALPS II experiment we aim to reach a higher sensitivity in order to increase the probability of detecting axions or axion-like particles.
The sensitivity gains for the ALPs particles compared to ALPS I are achieved by increasing the magnetic length, introducing a regeneration cavity and an improved detector system. The main sensitivity gain is due to the enhanced magnet length arising from 2 times 12 HERA-dipole magnet string. A sizable additional gain arises from the installation of the regeneration cavity.
The numbers given in this table are for a transition edge sensor detector, as this is the detector which is expected to be used in the final version of all stages of ALPS II. For hidden photons, there is no gain from the magnetic field. Thus, the sensitivity gain follows as above except for the factor coming from BL and amounts to 147 for hidden photons.
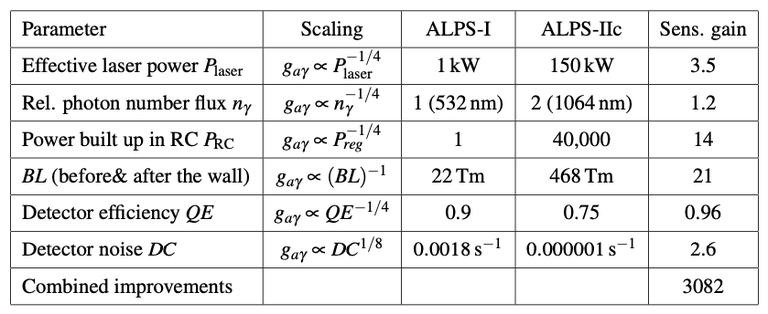
Parameters of the ALPS I experiment in comparison to the ALPS II. The second column shows the dependence of the reachable ALP-photon coupling on the experimental parameters. The last column lists the approximate sensitivity gain for axion-like particle searches compared to ALPS I.
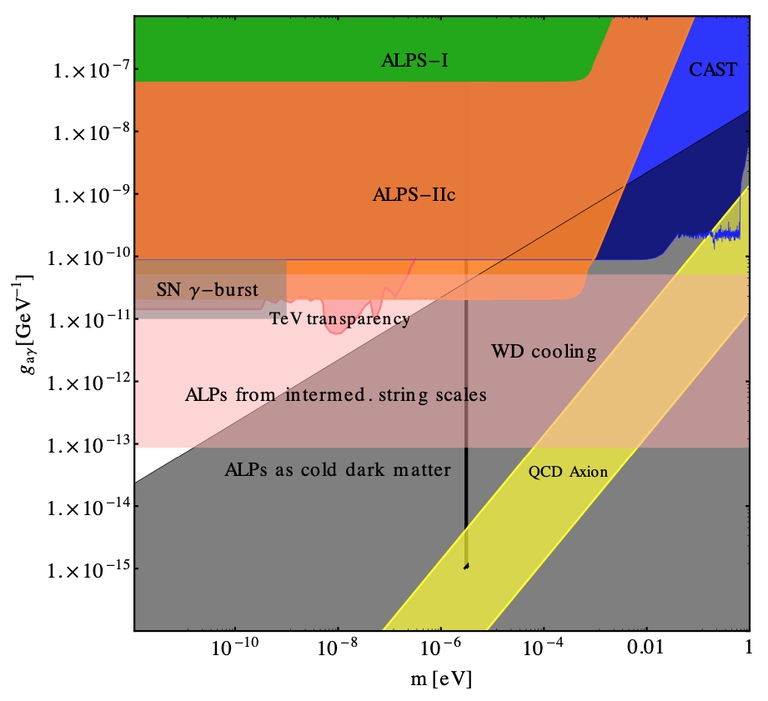
Schematic overview of the sensitivity reach of the final stage of ALPS II, ALPS IIc depicting its scientific impact.
The expected sensitivity of ALPS II experiment for the ALPs and hidden-photon search are compared to exclusion limits set by the other experiments. As a yellow band, generic QCD axion models are indicated. For comparison, the ALPS I results (in green, max. sensitivity 7 × 10−8 GeV−1 ) and the currently most stringent bounds on ALPs in this mass region from the CAST helioscope (in blue) are shown.
As visible, ALPS-IIc (final stage of ALPS II, in orange, max. sensitivity 2 × 10−11 GeV−1 ) will surpass the CAST bounds in the lower mass region and tackle parameter regions in which ALPs are motivated by fundamental particle physics questions (cold Dark Matter candidates, in gray, and predictions from string theory around 10−12 GeV−1 , not colored) as well as astrophysical hints: TeV transparency (red, fiducial region) and WD cooling (light red band)). In addition the parameter region excluded from the 1987 a super-nova burst is indicated. The predictions from theory and astrophysical considerations are generally certain within about an order of magnitude. Note that the parameter range between µeV < m < 1meV is particularly interesting in the context of axion Dark Matter, and currently most successfully probed by ADMX (black line). ALPS, on the other hand, can be Dark Matter in a much wider mass range.